What is the Modigliani Miller? The Modigliani–Miller theorem is an influential element of economic theory; it forms the basis for modern thinking on capital structure. Modigliani and Miller approach to capital theory, devised in the 1950s advocates capital structure irrelevancy theory. This suggests that the valuation of a firm is irrelevant to the capital structure of a company. So, what is the topic we are going to discuss; Understand the Modigliani Miller Proposition with the Capital Structure Theory!
Here are explained formula of the Modigliani Miller Proposition for the Capital Structure Theory!
The capital structure of a company is the way a company finances its assets. A company can finance its operations by either equity or different combinations of debt and equity. The capital structure of a company can have a majority of the debt component or a majority of equity or a mix of both debt and equity. Modigliani and Miller, two professors in the 1950s, studied capital-structure theory intensely. From their analysis, they developed the capital-structure irrelevance proposition.
Essentially, they hypothesized that in perfect markets, it does not matter what capital structure a company uses to finance its operations. They theorized that the market value of a firm is determined by its earning power and by the risk of its underlying assets and that its value is independent of the way it chooses to finance its investments or distribute dividends.
Modigliani and Miller’s Capital Structure Irrelevance Proposition:
The M&M capital-structure irrelevance proposition assumes no taxes and no bankruptcy costs. In this simplified view, the weighted average cost of capital (WACC) should remain constant with changes in the company’s capital structure. For example, no matter how the firm borrows, there will be no tax benefit from interest payments and thus no changes or benefits to the WACC.
Additionally, since there are no changes or benefits from increases in debt, the capital structure does not influence a company’s stock price, and the capital structure is therefore irrelevant to a company’s stock price. However, as we have stated, taxes and bankruptcy costs do significantly affect a company’s stock price. In additional papers, Modigliani and Miller included both the effect of taxes and bankruptcy costs.
Modigliani Miller Proposition:
The following Proposition is two types below are:
Proposition-I
The Modigliani-Miller Proposition-I Theory (MM-I) states that under a certain market price process, in the absence of taxes, no transaction costs, no asymmetric information and in a perfect market, the cost of capital and the value of the firm are not affected by the change in capital structure. The firm’s value is determined by its real assets, not by the securities it issues. In other words, capital structure decisions are irrelevant as long as the firm’s investment decisions are taken as given.
The Modigliani and Miller explained the theorem was originally proven under the assumption of no taxes. It is made up of two propositions that are (i) the overall cost of capital and the value of the firm are independent of the capital structure. The total market value of the firm is given by capitalizing the expected net operating income by the rate appropriate for that risk class. (ii) The financial risk increase with more debt content in the capital structure. As a result, the cost of equity increases in a manner to offset exactly the low-cost advantage of debt. Hence, the overall cost of capital remains the same.
The assumptions of the MM theory are:
- There is a perfect capital market. Capital markets are perfect when: 1) Investors are free to buy and sell securities. 2) Investors can trade without restrictions and can borrow or lend funds on the same terms as the firms do. 3) Investors behave rationally. 4) Investors have equal access to all relevant information. 5) Capital markets are efficient. 6) No costs of financial distress and liquidation, and 7) There are no taxes.
- Firms can be classified into homogeneous business risk classes. All the firms in the same risk class will have the same degree of financial risk.
- All investors have the same view for the investment, profits, and dividends in the future; they have the same expectation of a firm’s net operating income.
- The dividend payout ratio is 100%, which means there are no retained earnings.
In the absence of the tax world, base on MM Proposition-I, the value of the firm is unaffected by its capital structure. In other words, regardless of whether a company has liabilities, the total risk of its securities holders will not change even the capital structure is changed. As the weighted average cost of capital unchanged, so must the same as the total value of the company. That is VL = VU = EBIT/equity, where VL is the value of a levered firm = price of buying a firm that is composed of some mix of debt and equity, VU is the value of an unlevered firm = the price of buying a firm composed only of equity and EBIT is earnings before interest and tax. Whether or not the company has loans or the loans for high or low, investors are all accessible through the following two kinds of investment on their own to create the desired type of earning.
- Direct investments in the company’s stock borrowing
- If shares of levered firms are priced too high, investors will try to take advantage of borrowing on their own and use the money to buy shares in unlevered firms. The use of debt by the investors is known as homemade leverage.
The investors of homemade leverage can obtain the same return as the levered firms, therefore, for investors; the value of the firm is not affected by the debt-equity mix.
The MM Proposition I assumptions are quite unrealistic, there have some implications,
- Capital structure is irrelevant to shareholder wealth maximization.
- The value of the firm is determined by the firm’s capital budgeting decisions.
- Increasing the extent to which a firm relies on debt increases both the risk and the expected return to equity – but not the price per share.
Based on the inadequate of MM Proposition-I, Franco Modigliani and Merton H.Miller revised their theory in 1963, which is MM Proposition-II.
Proposition-II
The Modigliani-Miller Proposition II Theory (MM II) defines the cost of equity is a linear function of the firm’s debt/equity ratio. According to them, for any firm in a given risk class, the cost of equity is equal to the constant average cost of capital plus a premium for the financial risk, which is equal to debt/equity ratio times the spread between average cost and cost of debt. Also, Modigliani and Miller recognized the importance of the existence of corporate taxes.
Accordingly, they agreed that the value of the firm will increase or the cost of capital will decrease with the use of debt due to tax deductibility of interest charges. Thus, the value of the corporation can be achieved by maximizing debt component in the capital structure. This theory of capital structure for the study provided an important and analytical framework. According to this approach, value of a firm is VL = VU = EBIT (1-T) / equity + TD where TD is tax savings. MM Proposition II is assuming that the tax shield effect of each is the same, and continued insight.
Leverage firms are increased in interest expense due to reduced tax liability, has also increased the allocation to the shareholders and creditors of the cash flow. The above formula can be deduced from the company debt the more the greater the tax saving benefits, the greater the value of the company. The revised capital structure of the MM Proposition-II pointed out that the existence of tax shield in a perfect capital market conditions cannot be reached, in an imperfect financial market, the capital structure changes will affect the company’s value.
Therefore, the value and cost of capital of the corporation with the capital structure changes in different leverage, the value of the levered firm will exceed the value of the unlevered firm. MM Proposition theory suggests that the higher the debt ratio is more favorable to corporate, but through borrowing adds an interest tax shield it may lead to costs of financial distress. Financial distress occurs when promises to creditors are broken or honored with difficulty.
Financial distress may lead to bankruptcy. The trade-off theory of capital structure theory in MM based on the added risk of bankruptcy and further improves the capital structure theory, to make it more practical significance. A firm that follows the trade-off theory sets a target debt to value ratio and then gradually moves towards the target. The target is determined by balancing the tax benefits of using debt against the costs of financial distress that rise at an increasing rate with the use of leverage.
It so predicts the moderate amount of debt as optimal. But there is evidence that the most profitable firm in an industry tend to borrow the least, while their probability of entering in financial distress seems to be very low. This fact contradicts the theory because if the distress risk is low, an increase in debt has a favorable tax effect. Under the trade-off theory, high profits should mean more debt-servicing capacity and more taxable income to shield and therefore should result in a higher debt ratio.
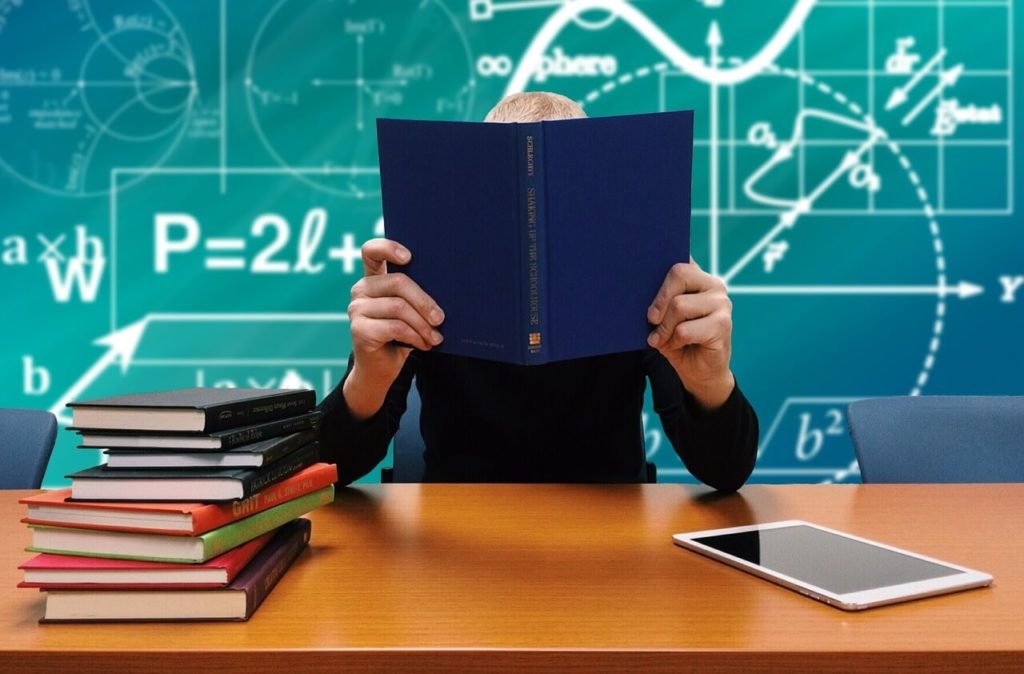
Understand the Capital Structure Decision in Corporate Finance:
Corporate finance is a specific area of finance dealing with the financial decisions corporations make and the tools as well as analysis used to make these decisions. The discipline as a whole may be divided among long-term and short-term decisions and techniques with the primary goal being maximizing corporate value while managing the firm’s financial risks. Capital investment decisions are long-term choices that investment with equity or debt, and the short-term decisions deal with the balance of current assets and current liabilities which is managing cash, inventories, and short-term borrowing and lending.
Corporate finance can be defined as the theory, process, and techniques that corporations use to make the investment, financing and dividend decisions that ultimately contribute to maximizing corporate value. Thus, a corporation will first decide in which projects to invest, then it will figure out how to finance them, and finally, it will decide how much money, if any, to give back to the owners. All these three dimensions which are investing, financing and distributing dividends are interrelated and mutually dependent. The capital structure decision is one of the most fundamental issues in corporate finance.
The capital structure of a company refers to a combination of debt, preferred stock, and common stock of finance that it uses to fund its long-term financing. Equity and debt capital are the two major sources of long-term funds for a firm. The theory of capital structure is closely related to the firm’s cost of capital. As the enterprises to obtain funds need to pay some costs, the cost of capital in the investment activities is also the main consideration of the rate of return.
The weighted average cost of capital (WACC) is the expected rate of return on the market value of all of the firm’s securities. WACC depends on the mix of different securities in the capital structure; a change in the mix of different securities in the capital structure will cause a change in the WACC. Thus, there will be a mix of different securities in the capital structure at which WACC will be the least.
The decision regarding the capital structure is based on the objective of achieving the maximization of shareholders wealth. With regard to the capital structure of the theoretical basis, the most well-known theory is Modigliani-Miller theorem of Franco Modigliani and Merton H.Miller. Yet the seemingly simple question as to how firms should best finance their fixed assets remains a contentious issue.
The Designing an Optimal Capital Structure:
The optimal capital structure refers to a proportion of debt and equity at which the marginal real cost of each available source of financing is the same. This is also viewed as a capital structure that maximizes the market price of shares and minimizes the overall cost of capital of the firm. Theoretically, the concept of optimal capital structure can easily be explained, but in operational terms, it is difficult to design an optimal capital structure because of a number of factors, both quantitative and qualitative, that influence the optimum capital structure. Moreover, the subjective judgment of the finance manager of the firm is also an influencing factor in designing the optimum capital structure of a firm. Designing the capital structure is also known as capital structure planning and capital structure decision.